Hydrogen will play a vital role in the deep decarbonization of the global economy. In a net-zero world, estimates like those provided by the International Renewable Energy Association (IRENA) and the Hydrogen Council forecast the chemical will supply between 12 and 20 percent of world final energy demand.
For this reason, hydrogen is gaining increased attention from policymakers and the public. While altogether a welcome development, it also comes with risks. The reason for this is simple: hydrogen is an energy carrier, not a primary fuel, and its production requires energy. For this reason, the process of devoting financial and clean energy resources to create hydrogen for applications where it is not optimal will detract from carbon abatement efforts, particularly when investments and energy are devoted to make clean hydrogen for applications which are more efficiently electrified.
The bottom of the ladder
Hydrogen is versatile, and its properties are ideal for the decarbonization of many vital economic processes which cannot easily be electrified, especially in industries such as refining, steel, and cement. Nonetheless, there are many applications which do not draw on hydrogen’s physical strengths, which policymakers should be wary of:
- Liquefaction for exports. Liquefying hydrogen will be too energy intensive per unit of energy, and therefore too expensive, to justify transporting the liquid product. Converting a fuel (wind, solar, water energy, natural gas, etc.) to electricity, from electricity to hydrogen, and then from hydrogen to liquified hydrogen will result in a roundtrip efficiency (the efficiency derived from dividing the energy carried in the primary fuel by the energy present in the resultant product) of less than 40 percent, even before factoring in boil-off and regasification. This figure decreases substantially if hydrogen imports are destined for electricity generation.
Compounding these disadvantages, the amount of energy which can be transported via hydrogen, even in liquid form, is 40 percent that of an equivalent volume of liquid natural gas.
Finally, the export and import of liquid hydrogen will largely not be able to repurpose existing liquefied natural gas (LNG) terminals. Hydrogen embrittlement will require new storage tanks, piping, pumps, and valves, while new, higher-capacity compressors will be needed to achieve a liquified temperature of −253 degrees Celsius, as opposed to −162 degrees Celsius for natural gas. These expensive and time-consuming refurbishments would severely harm project economics. - Baseload thermal power generation. It is unlikely that converting electricity or natural gas to hydrogen and then to electricity will be economically viable, except in limited circumstances, such as long-term storage. Utilizing hydrogen for power generation exhibits poor roundtrip efficiency. The maximum achievable roundtrip efficiency for power-to-power hydrogen applications is 29 percent, per a study published in the International Journal of Hydrogen Energy, and between 18 and 46 percent, per a study published in Nature Energy. For reference, a combined-cycle natural gas plant can operate at efficiencies ranging from 45 to 60 percent. Co-firing natural gas with hydrogen or using a 100-percent blend of hydrogen in gas turbines may have value for inter-seasonal storage applications, however.
- Domestic heating. Electrifying heat via heat pumps is highly efficient, while blending hydrogen into residential gas distribution pipelines for heating is far less so. In the United Kingdom, a meta-study by Oxford’s Jan Rosenow of thirty-two independent studies found that hydrogen for space and hot water heating results in higher energy system costs; leads to more significant environmental impacts, including greater land use requirements; and requires about five times more electricity to heat a home than a heat pump. Michael Liebreich, the founder of Bloomberg New Energy Finance, calculates that obtaining the equivalent of 70 gigawatts (GW) of residential heat via hydrogen would require 150 GW of renewable electricity. Conversely, obtaining that same amount of residential heat via heat pumps would require only 26 GW of renewable electricity.
A study on hydrogen by the UK Parliament found “hydrogen could play a role in domestic heating, but the extent of its potential is still uncertain and looks likely to be limited rather than widespread.” - Mid- to low-temperature industrial process heat. These applications should be electrified to the greatest feasible extent. Technologies like industrial heat pumps show considerable promise.
- Ground transportation (light transportation, trains, and buses). Batteries are more efficient, converting 80-90 percent of stored electricity to traction. This compares to 40-60 percent for hydrogen fuel cells, not accounting for the fact that hydrogen already needed to be converted from electricity or another feedstock. Batteries are also more advanced on the cost curve than fuel cells, will likely be able to deliver improved range, and enjoy incumbency advantages via more developed infrastructure. Moreover, in applications for these vehicles with fixed routes where fuel cells might make sense due to the availability of refueling infrastructure, electrical lines will be able to deliver better results at more competitive prices. However, hydrogen vehicles could play a pivotal role in certain heavy-duty markets, if batteries are unable to meet weight-to-volume requirements which enable them to power these vehicles as innovation progresses.
Key hydrogen applications
While hydrogen has limitations, it will have an important role to play in the decarbonization of key applications. Hydrogen burns at a higher temperature (2182 degrees Celsius) than natural gas (1937 degrees Celsius), yet combustion of hydrogen produces only water. Hydrogen is also an ideal solution for industrial applications such as steel, which is responsible for 7-9 percent of global greenhouse gas emissions.
- Chemicals. The first priority for building the market for low- to zero- carbon hydrogen should be to decarbonize existing hydrogen applications. This begins with refining—where hydrogen is used for hydrotreating and hydrocracking, which are essential to process heavy sour crude oil—and chemicals, with an emphasis on nitrogen-based fertilizer for which hydrogen is used to produce ammonia.
Today, the demand for hydrogen totals about 100 million tons per annum. Today, less than one million tons per annum are derived from “low-carbon” sources of hydrogen production, although this could scale to 16-24 million tons per annum by 2030 if all projects in the pipeline today were completed. - Steel. Hydrogen is essential to decarbonizing steel. Through a process called the direct reduction of iron, which uses hydrogen to reduce iron instead of coke, green steel can be made, bypassing the dirty blast-furnace-basic oxygen furnace (BF-BOF) process, in oversimplistic terms. While electrochemical substitute processes are on the horizon, they are not yet technologically mature.
- Long-duration energy storage. Unlike batteries, which store electricity electrochemically in a galvanic cell, hydrogen is a chemical energy storage solution which can play a role in integrating the electrical grid by storing energy for long periods of time, eventually being converted back to electricity via combustion or by using fuel cells. The use of hydrogen for short-term “grid balancing”—the production of hydrogen during periods of high electricity production for use when production drops—competes with batteries, which store energy better per unit of volume and are achieving manufacturing scale already. However, the production of hydrogen with the express intent to fill protracted gaps in firm electricity generation is more cost-effective than using valuable battery storage for this purpose. Batteries are more economically employed for short-term storage, balancing daily ebbs in renewable generation.
A mix of uncertainty and necessity
One area to watch is decarbonizing heavy-duty vehicles, such as for mining and construction applications, whose requirements may differ from long-haul trucking. The best pathway to decarbonize haul trucks, for instance, is uncertain due to the weight-to-volume ratio for batteries approaching the scale needed to power these applications. Companies such as First Mode are trialing hydrogen haul trucks for mine sites and have fielded models which deliver more power than diesel predecessors.
Other hard-to-abate sectors similarly pose substantial amounts of uncertainty, with no clear candidate for the best pathway for decarbonization. This includes aviation, where hydrogen may yet emerge as a viable pathway for enabling low-emission flights, at least in certain contexts. This also includes shipping, for which methanol—a hydrogen carrier—may be a leading solution.
In any case, universal electrification is not possible with currently available technologies. Hydrogen will remain an irreplaceable building block of a net-zero energy system, and policymakers should continue to value it as such. But everything that can be electrified should be, leaving clean hydrogen for the most economically viable and carbon-intensive applications, allowing us to accelerate deployment where it is most needed.
William Tobin is a program assistant at the Atlantic Council Global Energy Center.
Joseph Webster is a senior fellow at the Atlantic Council Global Energy Center.
Meet the authors
Related content
Learn more about the Global Energy Center
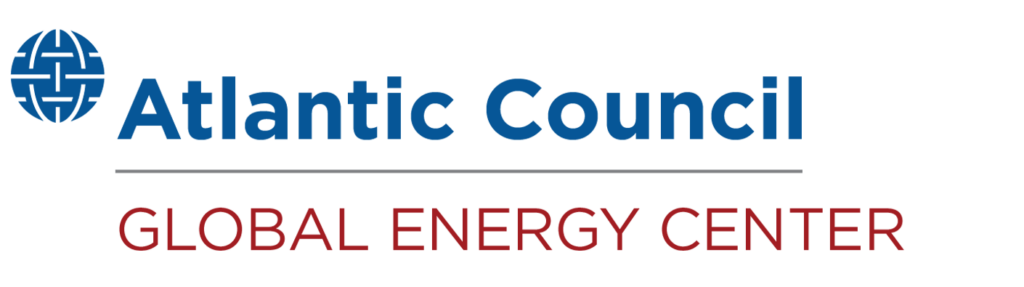
The Global Energy Center develops and promotes pragmatic and nonpartisan policy solutions designed to advance global energy security, enhance economic opportunity, and accelerate pathways to net-zero emissions.
Image: A wind farm in Germany. (Karsten Würth, Unsplash, Unsplash License) https://unsplash.com/license